Transcatheter aortic valve replacement (TAVR) is an innovative treatment modality for patients with severe symptomatic aortic stenosis (AS). After gaining initial market approval in Europe in 2007 and 4 years later in the US, TAVR continues to make large strides. An estimated 340,000 TAVR procedures have been completed in Europe in the immediate 5 years after approval and a further 10,000 procedures in the US were completed in the 2 years following approval in 2011.1 Global TAVR procedure volumes are expected to reach 300,000 annually, ushered in by the broadening of indications from everemerging data. Placement of Aortic Transcatheter Valve (PARTNER) I A and B were pivotal, initial trials, that established TAVR as an effective treatment for patients who were not surgical candidates.2–4 More recently, indications have continued to expand with the PARTNER II and Surgical Replacement and Transcatheter Aortic Valve Implantation (SURTAVI) trial results demonstrating TAVR as a non-inferior alternative to surgery in patients at intermediate risk, leading to US approval in this expanded population.5,6 TAVR is not without its complications; cerebrovascular accidents are one of the most important and clinically significant adverse events. Neuroprotection devices aimed at thwarting lesions, though promising, have yet to gain widespread clinical use. Establishing TAVR as the preferred treatment modality for severe symptomatic AS necessitates a more thorough understanding of iatrogenic stroke risk and measures to mitigate such complications.
Stroke Definitions
In the context of valve replacement clinical trials, three large research consortiums define stroke and provide guidelines for endpoint selection that are presented in Table 1: The Valve Academic Research Consortium (VARC)-2, American Heart Association/American Stroke Association (AHA/ASA), and more recently the Neurologic Academic Research Consortium (NeuroARC). Definitions for stroke and transient ischaemic attack (TIA) have matured with neuroimaging techniques and increased availability of MRI, focusing on tissue-based criteria instead of symptoms.7,8 The most recent publication from the AHA/ASA addressing stroke definitions placed an emphasis on CNS infarctions, which is defined in Table 1.8 Accordingly, neuroimaging is sufficient for identification of CNS infarction and stroke. In contrast, VARC-2 defines both disabling and non-disabling strokes primarily based on a clinical evaluation and scoring tool, the modified Rankin Scale (mRS) (Table 1).9 Comparatively, the AHA/ASA definition distinguishes sole CNS infarct from clinical stroke while VARC-2 does not define silent infarcts nor is there a distinction between sole CNS infarct from clinical stroke. The approach outlined by NeuroARC defines the full spectrum of cerebrovascular injury combining well-established symptom-based criteria with more sensitive tissue-based findings.10 A more comprehensive set of definitions should spur data acquisition allowing a distinction to be made between clinically meaningful and incidental findings. Accordingly, NeuroARC classifications fall into three major types: “overt (acutely symptomatic) CNS injury (Type 1), covert (acutely asymptomatic) CNS injury (Type 2), and neurological dysfunction (acutely symptomatic) without CNS injury (Type 3)”. The three major types can be further broken down into subtypes; however, for the purposes of our discussion, we present the most frequently encountered definitions in Table 1.
Peri-procedural Stroke Rates
The rate of combined stroke or transient ischaemic attack in early trials (PARTNER A) comparing TAVR to surgical aortic valve replacement (SAVR), was reported to be 5.5 % versus 2.4 % at 30 days (p=0.04) and 8.3 % versus 4.3 % (p=0.04) at one year, respectively.11 In PARTNER B, TAVR was compared to medical management for patients who were not suitable SAVR candidates: stroke was observed more frequently amongst TAVR patients at 1 year, 7.8 % versus 3.9 % (p=0.18).4 Importantly, there was no independent adjudication of stroke in the PARTNER I trials. Therefore, the observed difference in stroke rates and timings between surgery and TAVR must be considered hypothesis generating only. All other randomized trials point towards higher risk of stroke in SAVR compared to TAVR. For example, comparisons of Medtronic’s CoreValveTM to surgery observed all stroke less frequently in TAVR patients than in the surgical group at 2 years, 10.9 % versus 16.6 % (p=0.05).12 In a prospective, non-randomized, single-arm clinical study of CoreValve in patients at extreme surgical risk, combined stroke rates at 30 days and 1 year were reported to be 4.0 % and 7.0 %, respectively. More recently, SURTAVI results from an intermediate risk cohort found 4.5 % of patients in the SAVR group had a disabling stroke compared to the 2.6 % in TAVR group at 24 months.5 Thus, even among intermediate risk patients, stroke is a serious concern. The Society of Thoracic Surgeons (STS)/American College of Cardiology (ACC) Transcatheter Valve Therapy (TVT) registry captured 26,414 TAVR procedures as of December 2014. VARC defined, clinically adjudicated stroke rates were approximately 2 % in the STS/ACC TVT registry.13 Though the risk of stroke continues to decrease with advancements in valve technology, operator experience and patient selection, it remains a persistent and irrefutable reality.
The stroke incidence reported in these randomized approval TAVI trials represents only a small proportion of the totality of symptomatic stroke as they report major and disabling strokes. Indeed, stroke rates in aortic valve replacement studies vary based on stroke definition. Severe strokes rates, including major and disabling strokes, ranged from 1.6 % to 5.9 % (see Figure 1). Recent studies that include mild, moderate and severe symptomatic strokes, have reported total stroke rates ranging from 9 % up to 28 % with systematic evaluation by neurologists and neuroimaging in both SAVR and TAVR (see Figure 2).14–16 Variability in stroke incident rates is a factor of both differences in definitions and ascertainment method.
Stroke rates alone do not provide a complete assessment of periprocedural brain injury. Surrogate markers for clinical outcomes aid in device appraisal as CNS infarction can be acutely clinically silent and hence not meet criteria for stroke. Routine neuroimaging studies in patients undergoing TAVR procedures report that acutely silent ischaemic cerebral infarction caused by showers of cerebral emboli during valve instrumentation and placement is frequent. Volumetric analysis of infarcted brain tissue in these imaging studies ranged from 1.5 cm3 to 4.3 cm3, translating into a shocking estimation of 1 billion synapses and 2 million neurons.17 The implications linking acutely spontaneous silent CNS embolization to subsequent neurologic and cognitive impairment are immense, yet a gap in knowledge must be filled with respect to long-term neurologic and cognitive consequences of procedure-related iatrogenic cerebral embolization.18,19
Peri-procedural Stroke Timing
Increased risk for stroke following TAVR can be categorized into three distinct phases. The early phase, defined as the immediate 24 hours following a procedure, is most likely a direct result of the procedure and accounts for up 50 % of all cerebrovascular injury.20–23 Still, following TAVR, patients are at an increased risk during the delayed phase between days 2 and 30. Finally, stroke can occur up to 1 year following TAVR during the late phase. Late phase strokes may be attributed to patient comorbidities such as asymptomatic carotid stenosis or atrial fibrillation amongst other patient-related risk factors.9 In the PARTNER IA trial, within the randomized SAVR cohort, 62.5 % of the major strokes occurred within the first 2 days, 25 % between 5 and 30 days, and only 1 occurred after 30 days.24 In patients undergoing TAVR, a more significant proportion of early strokes occurred >24h after the procedure.25,26 Of the 11 post-procedure strokes in inoperable PARTNER patients, 27 % were within 24 hours, 55 % between 1 and 5 days, and 18 % after 1 week.4 Similarly, amongst a multicentre Canadian study, only 25 % of 30-day strokes were seen within 24h post-procedure.27 These statistics represent only clinically overt strokes and do not reflect covert strokes. Neuro-TAVI was a prospective study assessing neurologic complications in 44 patients. Of the 77.3 % of patients receiving diffusion-weighted MRI (DW-MRI) imaging, 94 % had a CNS lesion identified between post-procedure days 2 and 6. Pre-procedure MRI imaging was not performed and it was assumed that all lesions were new. At 30 days, 43 % of patients had NIH Stroke Scale (NIHSS) or Montreal Cognitive Assessment (MoCA) worsening.28 NeuroARC classifies neurological event timing into two categories: peri-procedural occurring up to 30 days post-intervention and late occurring any time after 30 days post-procedure. Accurate and meaningful stroke rates following valve implantation cannot rely solely on clinical symptoms, yet the clinical consequences of surrogate markers for infarct such as DW-MRI remain poorly defined. The distinct phases in which stroke occurs post-procedurally play a significant role in determining optimal timepoints for identifying CNS lesions in TAVR and neuroprotection trials.
Clinical Insight: Patient and Physician Perceptions
A peri-procedural stroke has calamitous clinical consequences for the patient. Peri-procedural stroke results in a five-fold increase in mortality.24,30 Furthermore, 40 % of survivors become permanently dependent, while a further 80 % experience social isolation and significant financial strain.31,32 It is therefore predictable that, when queried, a cohort of 785 patients viewed stroke as being 50 % to 250 % worse than death. Conversely, cardiologists viewed a patient’s death as being a worse outcome compared to stroke.33 Unfortunately, a disconnect exists between patient and physician perception that cannot be brushed aside.
Significance of Covert Stroke
Systematic studies in elderly patient populations without symptomatic cerebrovascular disease, notably the Framingham Study, Rotterdam Study, and Cardiovascular Health study, consistently identified MRI evidence of infarct.34–36 The Leukoaraiosis and Disability (LADIS) study, in elderly patients with minor neurocognitive symptoms and minor neurocognitive disability, identified MRI evidence of infarct to an ever greater extent.37 These significant findings cannot be written off as benign effects of ageing as there is evidence that covert MRI findings associated with subtle brain dysfunction are more prevalent than covert stroke.38,39 Correlating the wide array of neurologic findings with radiologic evidence in TAVR trials is an arduous task that can only benefit from accelerated, standardized data collection.
Stroke Mechanisms in the Context of TAVR
Early phase strokes are most likely related to procedural factors. Catheter manipulation within the aorta along with valve, catheter, and wire manipulation across the aortic valve are the most likely causes of procedural embolization.40–42 The transfemoral approach remains the preferred method for access, yet the STS/ACC TVT registry revealed that in 2013 the transapical approach, for a brief period, was used in more than 40 % of patients.13 A single-centre, prospective study comparing transapical to transfemoral access in 1000 patients found VARC-2-defined stroke rates to be comparable between the two approaches.43 Though access does not seem to play a part in stroke rates, in a study investigating use of a dual filter embolic protection device (SentinelTM), embolic debris was captured from 99 % of patients undergoing TAVR. Histological examination of the debris was significant for fibrin, amorphous calcium and connective tissue consistent with derivation from aortic valve leaflets or the aortic wall.44 In relation to TAVR, ischaemic stroke must be contrasted from global hypoxaemic injury. Ischaemic stroke always occurs in specific vascular territories, either arterial or venous, while global hypoxemic-ischaemic injury results in diffuse neuronal injury irrespective of vascular territory.45 Mortality rates for severe global hypoxic-ischaemic injury reach 80 % compared to <13 % with ischaemic stroke.46,47 Neuroprotection devices cannot be expected to impact global hypoxaemic-ischaemic injury and can only be beneficial for focal or multifocal ischaemic injury.
Selecting Neurologic Endpoints in Cardiovascular Trials
As stroke continues to be a serious peri-procedural complication of TAVR, it is important to collect accurate and meaningful data for device assessment. The most complete approach favours combining clinically validated screening tools, such as the NIHSS and MoCA, with sensitive imaging methods, such as DW-MRI.
Radiologic Guided Endpoints in Cardiovascular Trials
Ischaemic CNS tissue changes can be identified by DW-MRI within minutes to days.48,49 A Cochrane review comparing CT and MRI to the clinical diagnosis as a reference found DW-MRI to be significantly more sensitive than CT imaging.50 MRI is thus the preferred imaging modality for detection of CNS ischemia (see Figure 3). Accurate quantification of brain ischaemia further contributes to the utility of DW-MRI. Lesion volumes are maximal 5–7 days following injury, and can change 1–3 weeks after injury.51,52 NeuroARC recommends routine MRI imaging at 2–7 days following a procedure and non-routine MRI imaging if neurologic symptoms or delirium develop. Routine MRI endpoints should include Total Lesion Volume (TLV) (mm3).10 In a study comparing 32 TAVR patients to a historical cohort of 21 patients undergoing SAVR, 84 % of the TAVR patients had new DW-MRI lesions versus 48 % in the SAVR cohort. Both at the time of imaging and 3-month follow-up, there were no detectable cognitive defects when assessed by Mini Mental Status Exam (MMSE) or NIHSS.44 In a prospective study of 44 TAVR patients, DW-MRI lesions were detected in 94 % of patients, but neurologic impairment detected by NIHSS worsening occurred in only 22.6 % of patients at discharge and 14.8 % of patients at 30 days.28 As DW-MRI imaging is one of the most sensitive radiographic imaging modalities, there may be a poor correlation between DW-MRI endpoints and clinical symptoms in early trial phases. The goal of acquiring routine radiographic evidence is to make correlative assessments at later stages of trials, weeks and years after valve implantation.
Clinical Evaluations as Endpoints in Cardiovascular Trials
The tools available for clinical assessments of neurologic and functional impairment along with cognitive ability are numerous. In the acute setting of a stroke, the NIHSS, first described by Brott et al. in 1989, is a well-established and highly validated tool for assessing the severity and prognosis of a stroke.53–56 The NIHSS can be used in trials to routinely screen patients following cardiovascular procedures. Long-term stroke outcomes are assessed with the modified Rankin Scale (mRS) at 90 days to classify adverse events as disabling or non-disabling.57,58 Pre-procedural assessments of stroke, disability, delirium, cognition and quality of life should also be made and can be supplemented by baseline MRI imaging. Following a procedure, stroke, disability, cognition and quality of life should be assessed after 30–90 days and 1 year. A battery of tests is available to assess cognition.10 The Montreal Cognitive Assessment (MoCA) is commonly used as a screening, royalty-free clinical tool to detect frank cognitive changes.59 Interobserver variability in some tests is a real concern; however, most tests use standardized forms or calculators that reduce variability. With more routine acquisition and reporting, MoCA scores across multiple timepoints can be used to draw long-term correlations between DW-MRI acquired TLV and cognition.
Neuroprotective Devices
Currently, four embolic protection devices exist that have been previously described; two of which are deflectors and two of which are filters. These devices vary by access, valve access, position, coverage area, sheath size, and pore size (μm) but all ultimately attempt to prevent embolic debris during valve implantation from reaching cerebral circulation.60 A meta-analysis of five randomized clinical trials including 625 patients, combining CLEAN-TAVI, DEFLECT III, EMBOL-X, MISTRAL-C, and SENTINEL studies, and using death or stroke as a composite endpoint, found that neuroprotective devices reduced absolute risk by 4 % for a NNT of approximately 22.61 It is difficult to assess the significance of this meta-analysis as there is extensive heterogeneity in the studies due to various valves, neuroprotective devices, MRI scanners (3T versus 1.5T), patient risk and operator experience.
SentinelTM (Claret Medical)
MISTRAL-C was a hypothesis generating study randomizing TAVR patients to receive the Sentinel Cerebral Protection System (CPS). In this small trial with 32 patients in the device arm and 33 in the control arm, neurocognition was protected in the device arm when assessed by MMSE and MoCA at 5±1 days and there was also a decrease in number and volume of new MRI lesions.62 MoCA and MMSE testing at such an early time is not reflective of long-term consequences. Following MISTRAL-C, the Claret MontageTM Dual Filter System was studied in CLEAN-TAVI where the device was found to reduce the volume and size of new brain lesions on MRI 2 days post-TAVR; however, the stroke rate was similar between the intervention and control groups.14 In the SENTINEL trial, one of the most recent and largest studies to date, Kapadia et al. shed valuable light on the status of cardioembolic protection. In a 1:1:1 ratio, patients were randomized to an imaging control arm, imaging device arm, and a safety arm. DW-MRI did not detect a significant reduction in median total new lesion volume at 2–7 days after TAVR. Importantly, neurologic and neurocognitive function at 30 and 90 days were not significantly different either between the device and control group, although debris was found within filters in 99 % of patients.63 On the basis of the totality of data, the Claret device recently gained FDA approval in the US.
Embrella (Edwards Lifesciences)
PROTAVI-C was a pilot study for the Embrella deflector in which Rodés- Cabau et al. enrolled 54 patients, 12 of whom were controls and 42 receiving the device. Though DW-MRI detected lesions in both groups at 7 days after TAVR, the lesion volume was lower in the group receiving the device. Notably, there were statistically significant mild improvements in MoCA scores at 30 days in the device arm, but the control group also experienced MoCA improvement, albeit statistically insignificant. MMSE scores remained relatively unchanged for both groups from baseline and 30 days post-procedure.64 In a restrospective analysis of Embrella in which 15 patients received the device compared to a historical cohort of 37 patients who did not receive the device, Samim et al. found higher rates of ischaemic lesions in DW-MRI (9.0 versus 5.0, p=0.044) with Embrella, and this device is no longer under clinical evaluation.65
Embol-X (Edwards Lifesciences)
The Embol-X device was developed for use in open-heart surgery and requires direct access to the aorta. Modification of the device allowed it to be successfully used in three initial case reports for technical success and safety.66,67 Mack et al. recently presented results of a study investigating Embol-X, an embolic protection cannula positioned in the ascending aorta, and CardioGard, an intra-aortic filtration device, in SAVR patients. The trial was stopped early due to an interim analysis for futility. Though the devices captured debris in most patients, freedom from clinical or radiographic CNS infarction, the primary endpoint, was not observed.68
TriGuardTM (Keystone Heart Ltd)
DEFLECT I was a prospective, multicentre study aimed to evaluate safety and performance, in which 37 patients were enrolled. Post-procedure DW-MRI demonstrated that the presence of new cerebral ischaemic lesions was similar to historical controls (82 % versus 76 %, p=NS). It was further found that per-patient total lesion volume was 34 % lower when compared to the historical control (TLV 0.2 versus 0.3 cm3). Patients with complete cerebral vessel coverage experienced 89 % lower TLV compared to incomplete cerebral vessel coverage. With a MoCA score of 26 as the threshold for impairment, impaired patients had higher total median lesion volumes (163.18 cm3) compared to unimpaired patients (130.05 cm3).15 DEFLECT III was a multicentre, prospective, randomized study of TriGuard HDH Embolic Deflection Device in 85 patients randomized to TAVR with TriGuard or TAVR alone. Per-treatment population analysis revealed that TriGuard use was associated with greater freedom from new ischaemic brain lesions (26.9 % versus 11.15 %) and fewer neurologic deficits assessed by NIHSS (3.1 % versus 15.4 %). In terms of neurocognition, TriGuard use resulted in improved MoCA scores at discharge and 30 days along with better performance on a delayed memory tasks (p=0.028) at discharge.15 Long-term neurocognitive performance data, with a follow-up >1 year, is not available for any studies. The REFLECT US IDE trial is currently ongoing.
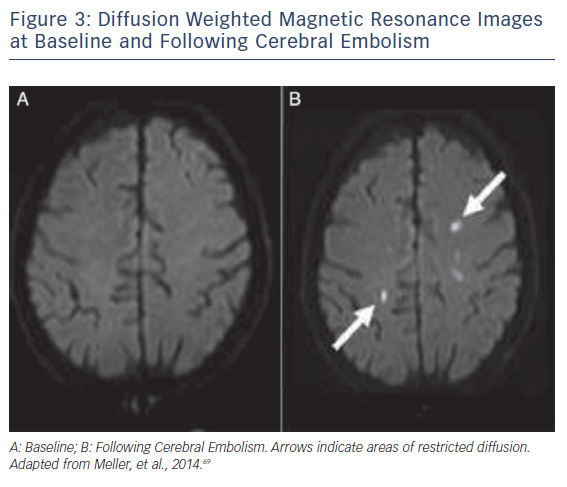
Conclusion
TAVR continues to evolve with the expansion of indications in intermediate risk patients. With the imminent inclusion of low-risk patients, cerebral infarction during TAVR may play a larger role in patients who presumably have more to lose. Despite the gravity and widespread prevalence of cerebral embolization during TAVR, there is a lack of clinical data to fully understand the long-term consequences of covert CNS infarction. Cerebral embolization during TAVR is a certainty, yet the uncertainties lay in demonstrating the clinical benefits of cerebral embolic protection and determining the optimal study design for evaluating devices aimed at mitigating risk. These methodological limitations and gaps in evidence linking acutely silent CNS infarction with longer-term neurologic and cognitive effects have led to delays in adopting cerebral protection. While short- and long-term clinical evidence continues to evolve and in the absence of a safety hazard, demonstrating a reduction in the extent of CNS infarction (irrespective of symptoms) should be sufficient burden of proof for cerebral embolic protection.